In the last blog I looked at the magnitude of the effect of aging on athletic performance, today I examine *why* fitness declines with age. In the next blog, I will examine how to minimize or reverse this!
We can divide this discussion into three major areas: your genes / your muscle mass and your metabolism……but let’s start with the big question why do we age at all?
Why Do We Age?
Aging takes place in every living creature basically one cell at a time. In short, if your cellular DNA damage (eg from oxidative stress) is greater than your cellular repair (eg from stem cells) then your organs will deteriorate and eventually something (eg your heart) will fundamentally break……and you will die! Sorry, I hope this does not come as a shock!
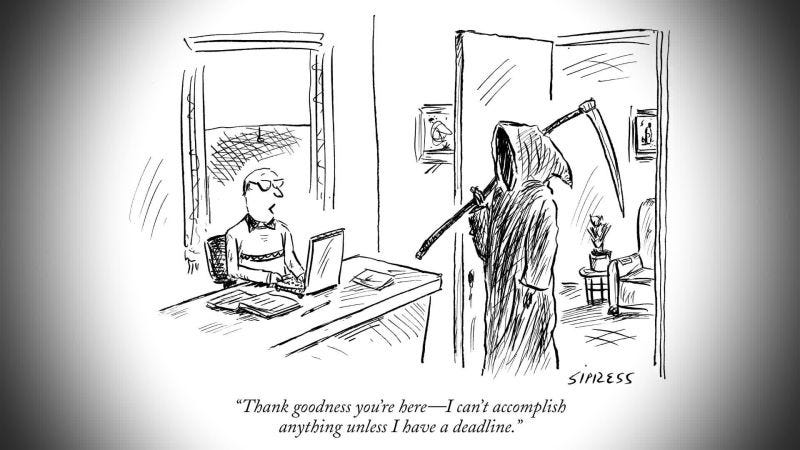
Every living thing is subject to the same limitation of aging cells (except perhaps these which might have tricked nature?? https://www.science.org.au/curious/earth-environment/animals-can-live-forever).
In fact lifespan itself is reasonably predictable across the animal kingdom, with three major traits having a strong influence:
brain weight, body weight, and resting metabolic rate.
In fact, there is an equation as follows: life span = 5.5E ^0.54S ^−0.34M ^−0.42 where E=brain weight S= body weight in grams and M= metabolic rate kcal/g/hr
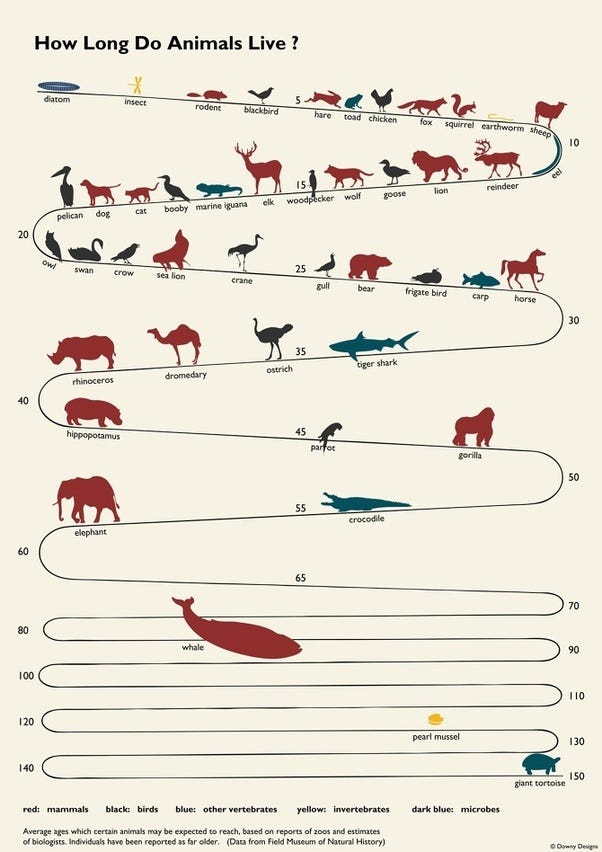
In humans, many insurance companies try and estimate life expectancy using a few headline factors like this one.
.So if aging is essentially a process when mechanisms of repair slow down below the rate of cellular damage then the aging process probably depends mostly on our ability to repair damaged chromosomes in cells, right? Indeed, we can get a measure of this in a few ways, such as stem cell activity.
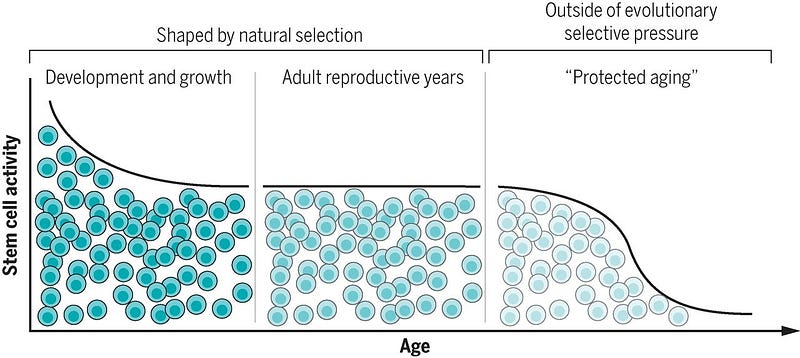
For example, neural stem cells are important for ongoing generation of new neurons in specific regions of the brain but play a limited role in damage repair. Muscle stem cells (or satellite cells) play a small role in muscle maintenance but vigorously engage in regeneration after injury. Hematopoietic stem cells and intestinal stem cells do both, contributing to on going production of differentiated cells and also repairing tissue.
In the early years of growth, stem cells are highly active and contribute to tissue formation. During the reproductive phase of life, stem cells maintain and repair tissues. But beyond reproductive maturity (when cell and tissue functions are predicted to be under little evolutionary pressure): stem cell functionality declines.
OK, that seems clear, but why does cellular damage occur in the first place? This is sadly a legacy of using oxygen as a fuel (evolution kick started this in eukaryotes). Oxygen is highly energetic which can be a good thing but also dangerous. The rise of oxygen concentrations in the Earth’s atmosphere and oceans was one of the most important events in life history, and is thought to have triggered the origin of eukaryotic life (and sexual reproduction!).
Many oxidative reactions (loss of electrons) take place within cells and result in highly reactive “free radicals” and leading to damage of cell components such as membranes (a theory first proposed by Denham Harman). Mutation of mitochondrial DNA impairs the function of proteins in the organelle’s respiration machinery, enhancing the production of DNA-damaging oxygen radicals, increased expression of proinflammatory genes, tissue inflammation and ultimately dysfunction and degeneration.
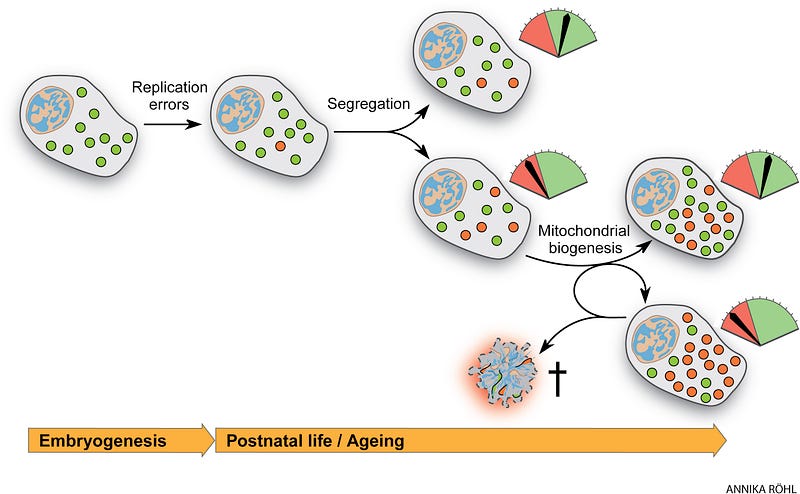
Mutations accumulate in stem cells, contributing to the age-associated decrease in homeostasis and regenerative potential. These are replication errors made by the mtDNA polymerase and oxidative damage. During the lifetime of an individual, both inherited and de novo mutations formed early in life can clonally expand, reflected in the mosaic nature of respiratory chain dysfunction observed in aging tissues. Accumulating evidence from the last three decades shows that mtDNA mutations, be they point mutations or large-scale deletions, increase with age both in humans and mammalian model organisms.
So that’s my attempt at explaining aging. Now let’s look at three big mechanisms of aging: genes / muscles / metabolism (mitochondria).
Mechanisms #1: Your genes (Telomeres)
One major contribution to aging is genetics. For example identical twins have life spans more similar in length than do non-twin siblings. By indeed looking at twins, it was estimated that lifespan could be about 12%-25% genetic. 30% of longevity variation in female mice is genetically determined (for males its 20%). Long lived people tend to have more ‘T’s in the single nucleotide polymorphisms FOXO3 gene‘rs7412,’ less ‘C’s in the cholesterol related APOE gene ‘rs429358’, and more ‘G’s in ‘rs2802292’.
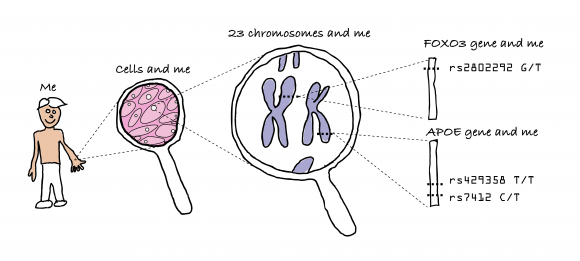
Fifty years ago, Leonard Hayflick and Paul Moorhead, discovered that many human cells have a limited capacity to reproduce themselves in culture by dividing. They found that after 40 and 60 cell divisions, but then can divide no more and this has been called the “Hayflick Limit”. It seems that what determines the Hayflick Limit for dividing human cells is the length of a cell’s telomeres. These are repeated segments of DNA on the ends of chromosomes.
You can therefore measure longevity using telomeres. The number of repeats in a telomere determines the maximum life span of a cell, since telomeres lose a little bit of their length during each cell division. Since replicative DNA polymerases are not able to replicate telomeres, and telomerase (specialized DNA polymerase that could replicate telomeres) are not expressed in normal mammalian somatic cells, telomeres become too short to replicate after a fixed number of cell divisions. Eventually, the cell will stop dividing and die.
Research has shown that telomeres are vulnerable to genetic factors that alter an organism’s rate of aging. In humans, variations in a gene known as TERC has been linked with increased rate of biological aging.
We can actually count up the telomere base pairs. We are born with telomeres between 5–12,000 base pairs long ; whereas mice have about 50,000 base pairs….. but they lose 7,000 base pairs per year compared to 15–50 base pairs per year in humans. A greater rate of telomere shortening therefore suggests a faster pace of ageing.
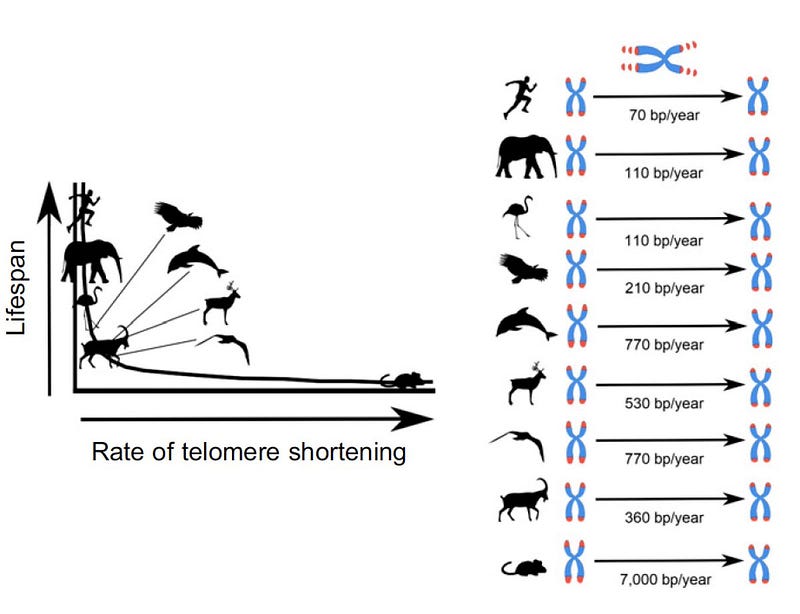
However, your body will respond to exercise, consider these two studies:
In a study of 2401 twins (LINK Arch Intern Med . 2008 Jan 28;168(2):154–8) researchers found study participants who spent more than 3 hours each week engaged in vigorous physical activity (such as lifting weights) had longer telomeres than subjects 10 years younger, suggesting that individuals who eschew placing a vigorous load on their body may wind up biologically older by 10 years.
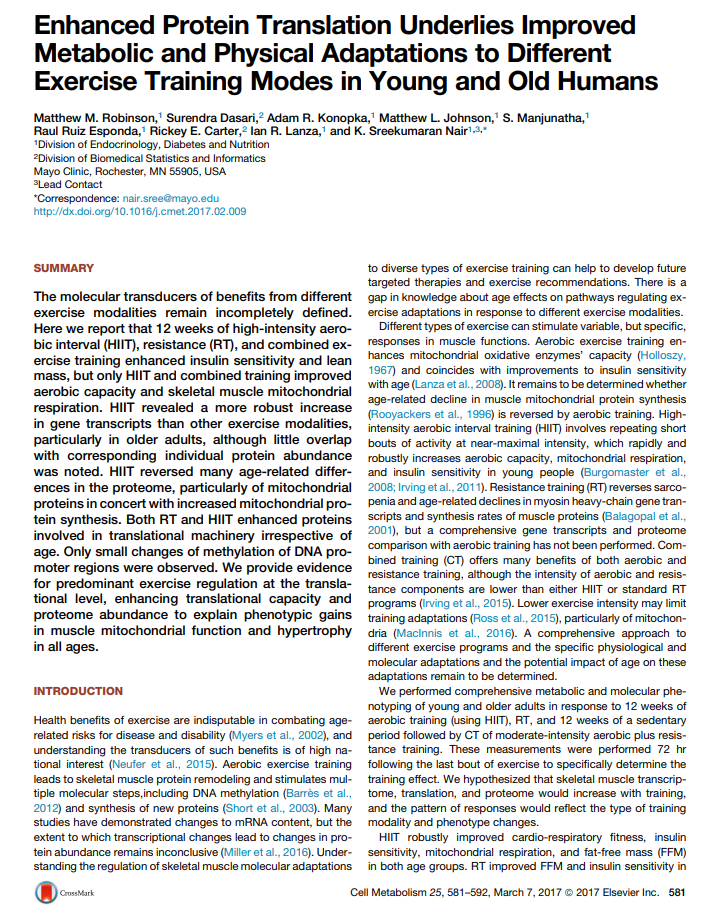
A 2nd study from Mayo Clinic compared groups of younger (18–30 years old) and older (65–80 years old) adults who did one of three kinds of exercise regimes for 12 weeks: resistance (strength building) exercises; high intensity interval training on stationary bikes ; or a combination of light resistance training and moderate-paced cycling [LINK]. Older participants experienced dramatic improvements at a cellular level. Scientists found that after just 12 weeks, almost 400 genes in the older participants who did HIT training on bikes, were working differently. In the younger group, only 274 genes changed. In the older group, a change was seen in only 33 genes for the weight lifting group and only 19 genes in the moderate exercise group.
Mechanism #2: Your Muscle Mass
Another big factor behind aging is muscle size (and muscle efficiency); ie a reduction in muscle mass as we get older. Muscles get thinner and weaker BUT this effect is massively influenced by exercise. After the age of 30, adults typically lose up to 10 oz. of muscle mass per year, just over 8 lbs. per decade (Evans & Rosenberg, 1991). This is due to both atrophy of exercise muscle fibers, and to degeneration of spinal motor neurons, usually the type II, fast twitch units. Many adults lose 30% of their muscle cells between the ages of 20 to 70 (so sarcopenia begins at 20! if you stop sports then).
Also with age, the number of cross-linkages within and between collagen molecules increases, leading to crystallinity and rigidity, which are reflected in a general body stiffness. There is also a decrease in the relative amount of hexosamine–collagen ratio, which has been investigated as an index of individual differences in the rate of aging.
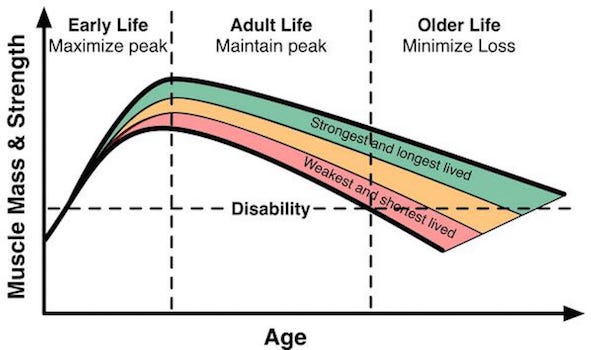
But again, exercise reduces this decline and both endurance (cardiovascular) and strength (resistance) training can help (see Solberg and Bouaziz).
Mechanism #3: Metabolism (Mitochondria)
Loss of muscle mass above is not a very informative answer on its own. We need to understand what underlines loss of muscle mass. The answer is mainly a decline in muscle mitochondrial protein synthesis (and mitochondrial gene expression). In one study, skeletal muscle demonstrated higher (40%) mitochondrial protein synthesis rate in 24 year olds compared with middle aged and older [ref].
Again, the good news is exercise training is mitoprotective and anti-sarcopenic. Exercise, both in the forms of endurance and resistance training, improves ETC electron flux and mitochondrial oxidative coupling. Specifically, resistance training increases the ratio of complex IV/complex I + III, which in turn minimizes electron leakage and thus ROS generation from complexes I and III, the main cellular sources of superoxide. At the level of mtDNA, this decreases oxidative damage of mtDNA and reduces prevalence of deletion-mutations.
In addition, when resistance training is coupled with endurance training, or with endurance training alone, mtDNA abundance, mitochondrial protein synthesis, and mitochondrial biogenesis all increase. At the level of muscle fibers, these exercise-induced changes prevent age-associated aberrant COX−/SDH++ phenotypes and preserve type II muscle fibers, altogether reducing muscle wasting with age (aka sarcopenia.) link for more info.
Conclusion & Summary
Aging is a consequence of cumulative damage in all cellular constituents especially DNA often from oxygen itself. Every day there are thousands of insults that affect DNA, including endogenous factors (such as metabolism) and exogenous factor (like diet, radiation, toxic substances). Each of the ~1013 cells in the human body receives tens of thousands of DNA lesions per day, the most pervasive environmental DNA-damaging agent is ultraviolet light (UV) where residual UV-A and UV-B in strong sunlight can induce ~100,000 lesions per exposed cell per hour.
Only a minimal amount (less than 0.02%) accumulates as permanent damage, while the rest is miraculously repaired by hundred of mechanisms. However, if only one important gene is not repaired and its function is vital such as a tumor suppressor then this could result in chromosomal aberrations causing certain cells to transform into cancer cells (link).
As Benjamin Franklin said “Nothing is certain except death and taxes“. However, that does not mean longevity occurs at a fixed rate. What you do now makes a big difference. Try that life expectancy calc again! Now we understand a lot of the mechanisms, we will look at what specific exercises we should undertake in the next blog.
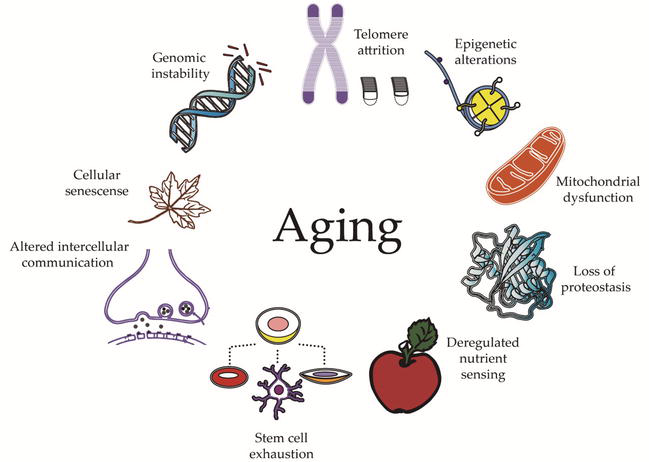
Citations
https://www.bioscience.org/2018/v23/af/4657/fulltext.htm
https://www.the-scientist.com/features/how-muscles-age–and-how-exercise-can-slow-it-64708